When the American Association for Cancer Research (AACR) Annual Meeting featured a plenary session focused on metastasis in 2010, our understanding of the complex process was far different than it is today.Â
“Back then, our textbook understanding of metastasis was … a linear process of obligate steps,” said Cyrus M. Ghajar, PhD, of Fred Hutchinson Cancer Center, who chaired a new metastasis-focused plenary session at the AACR Annual Meeting 2024, held in San Diego April 5-10. Â
Ghajar explained that our understanding has evolved—in the same way that metastatic tumor cells must evolve to conquer the challenges that underlie the process of traveling to a new location and thriving at a distant site. Metastases must adapt to their new surroundings, which can induce widespread changes to gene expression and metabolism. They also must influence their local microenvironment—as well as other organs—to further support their growth.Â
The ability of metastatic cells to evolve and adapt to ever-changing circumstances formed the core of the plenary session, “Evolution of the Genome, Microenvironment, and Host through Metastasis,” in which experts discussed how unique characteristics contribute to the sneaky, evasive nature of these microscopic colonizers.Â
A Seed is SownÂ
“If you can look into the seeds of time / And say which grain will grow and which will not / Speak then to me.”Â
This quote from William Shakespeare’s Macbeth was the response given to Christoph A. Klein, MD, PhD, from the University of Regensburg in Germany, when he proposed to his mentor the idea of using single-cell analysis to study the origins of metastasis nearly 30 years ago. Indeed, researchers at the time were already exploring ways to target the metastatic “seed” cells that break off from a primary tumor with the potential to form distant colonies.Â
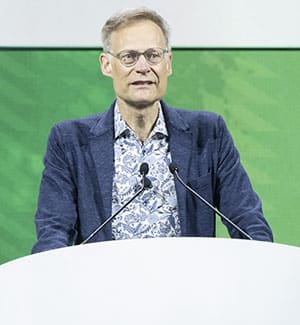
But newer research has shown that our understanding of metastases as subclonal populations that originate from the primary tumor is potentially misguided. Single-cell lineage analysis of solid tumor cells and disseminated cancer cells (DCCs) isolated at multiple time points from a patient with breast cancer revealed that the patient’s metastasis shared clonal characteristics with a DCC identified seven years before a diagnosis of metastatic disease; it did not, however, share a lineage with the primary tumor.Â
“This indicates that the primary tumor and the metastasis develop in parallel,” Klein said.Â
In another analysis, Klein and colleagues found that neither the number nor characteristics of DCCs from patients with overt metastasis could predict patient outcomes; however, the presence of stemlike DCCs—those predicted to become metastatic seed cells—in patients whose tumors had not yet metastasized correlated strongly with a poor prognosis.Â
The researchers searched for markers of this stemness phenotype and found a tyrosine kinase called c-KIT with elevated expression in stemlike DCCs; in publicly available data sets, high levels of c-KIT in DCCs correlated with poor survival, but high levels of c-KIT in primary tumors had no effect on patient outcomes. Klein expressed hope that developing therapies to target c-KIT may help destroy these metastatic seed cells before they pose a threat.Â
“If we want to target the metastatic seed—for example, in our adjuvant therapy settings—maybe now we have new markers,” Klein said. “c-KIT is a drug target—you have small molecules, you have [antibody drug conjugates], you can generate CAR T cells.”Â
A Seedling Takes RootÂ
The metastatic seed cells that colonize distant sites face significant hurdles on their journey, including survival in harsh environments and adaptation to new organ locations.Â
“The cell needs to go to a distant, distinct organ and be able to grow and thrive there in an environment that’s drastically different from its own. In fact, colonization is not one, but many hurdles,” said Dana Pe’er, PhD, of the Memorial Sloan Kettering Cancer Center and the Howard Hughes Medical Institute.Â
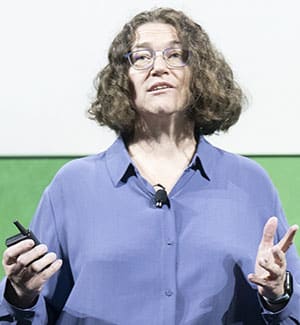
How does a single tumor cell evolve to conquer all these challenges? Pe’er emphasized that the trick lies not in tackling each challenge independently but rather upregulating gene programs related to plasticity—the cell’s ability to adapt to unexpected environmental changes.Â
Pe’er showed that primary tumor cells are enriched in transcriptional programs one might expect to be associated with plasticity, including stemness markers and genes involved in the epithelial-to-mesenchymal transition (EMT). Metastatic cells, however, expressed noncanonical plasticity programs. Pe’er and colleagues found that these noncanonical programs allowed tumor cells to revert to a fetal gene expression state before transforming into metastatic seed cells.Â
She used leptomeninges as a prime example of a hostile organ site wherein the survival of metastatic cells is somewhat surprising. Pe’er and colleagues found that one particular adaptation—the ability to thrive in low-iron environments—gave the metastatic cells the necessary tools to colonize the leptomeninges. The researchers have launched a phase I clinical trial evaluating whether leptomeningeal delivery of the iron chelator deferoxamine may help treat these metastases.Â
High plasticity means that metastatic cells may be better equipped to dodge additional hurdles, such as treatments, than primary tumors. “Metastases have to be targeted differently,” Pe’er said. “What we need to do is understand this plasticity—understand its causes—in order to be able to target the plasticity itself.”Â
A Sapling Soaks Up NutrientsÂ
Part of many metastatic cells’ innate adaptability is the power to leverage different metabolic pathways to feed their growth. Sarah-Maria Fendt, PhD, a researcher at the VIB Center for Cancer Biology and KU Leuven in Belgium, and winner of the AACR Award for Outstanding Achievement in Basic Cancer Research, explained how some metastases can take advantage of unique metabolites found in the lung microenvironment.Â
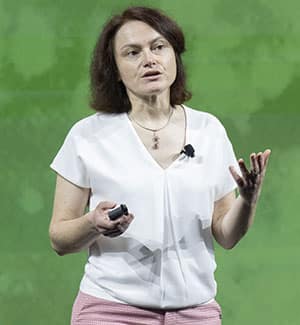
Fendt and colleagues observed that mice injected with cancer cells alone formed fewer metastases than mice that were given a milieu of tumor-secreted factors before adding cancer cells. The factors, Fendt explained, helped prime the future metastatic sites for better reception and growth.Â
When the researchers analyzed the gene expression of cells that grew in primed mice versus unprimed mice, they saw that metastases from primed mice had upregulated protein translation. Specifically, they found increased activity—but not expression—of the translation initiation factor eIF5A.Â
They also analyzed the lung interstitial fluid of primed and unprimed mice and found that lungs of primed mice had an abundance of aspartate. How, if at all, was this connected to the hyperactivation of translation?Â
Fendt and colleagues found that aspartate was not being taken up by metastatic cells but was instead binding to and activating N-methyl-D-aspartate (NMDA) receptors on their surface. Stimulation of NMDA boosted the cells’ ability to add hypusine residues to certain proteins, including eIF5A, which is activated by hypusination.Â
To validate this in humans, Fendt and colleagues analyzed rapid autopsy samples from individuals with and without breast cancer. Patients with breast cancer had significantly higher concentrations of aspartate in their lung interstitial fluid and evidence of upregulated translation in metastases.Â
Fendt noted that inhibitors of NMDA are already approved for clinical use. “We would like to propose that this can actually immediately help breast cancer patients,” she said. “These inhibitors that are currently used for the treatment of neurological disorders could be repurposed to treat breast cancer patients.”Â
A Tree Spreads its BranchesÂ
As Fendt explained, primary tumors can secrete soluble factors that help prime distant sites for future metastasis. These soluble factors include extracellular vesicles (EVs)—tiny lipid bubbles that can contain tumor-derived DNA, RNA, and proteins—said David C. Lyden, MD, PhD, of Weill Cornell Medical College.Â
But these vesicles don’t only travel to future metastatic sites. Some also travel to seemingly unrelated organs to assist the metastatic process in systemic ways, Lyden said. The full-body impact of such reprogramming can exacerbate symptoms, decrease drug efficacy, or even cause diseases beyond cancer.Â
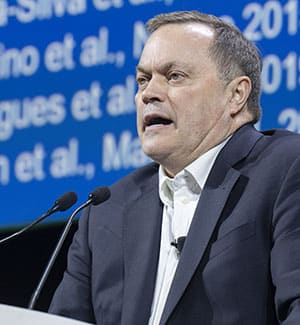
“These EVs can affect a wide range of systemic effects of cancer, such as cachexia or depression,” Lyden said. “It really can dysregulate the immune system—how are immunotherapeutics going to work if the tumor exosomes are taken up by these immune cells?”Â
For instance, Lyden and colleagues examined the EVs released by gastrointestinal tumors, such as pancreatic cancer, that often metastasize to the liver. While larger EVs called exosomes traveled to the liver to promote metastasis, smaller EVs called exomeres traveled to the lung, where they promoted the formation of dangerous blood clots—a condition called thrombosis.Â
Likewise, in lung-metastatic melanoma, Lyden and colleagues found that EVs promoted the dysfunction of a variety of systems. Some exosomes primed the lung for metastasis, other exosomes were taken up by immune cells, and exomeres invaded the liver, where they caused significant metabolic changes, including fatty liver disease.Â
“If you have cancer going to the lung, you can’t ignore that you generate a fatty liver—you can’t metabolize chemotherapy well … T cells don’t function, and you can really enhance cardiovascular disease,” Lyden said.Â
Lyden and colleagues are working on systemic strategies to not only block metastatic priming by these EVs but also dysregulation of other organs. For instance, they found that blocking tumor necrosis factor alpha (TNFα) in mice with EV-induced fatty livers could decrease fat deposition.Â
“The goal here is not only, in a cancer patient, to treat the primary tumor and the future metastatic organ. We must target the other niches involved in systemic effects of cancer,” Lyden said.Â